Protein Degradation During Exercise
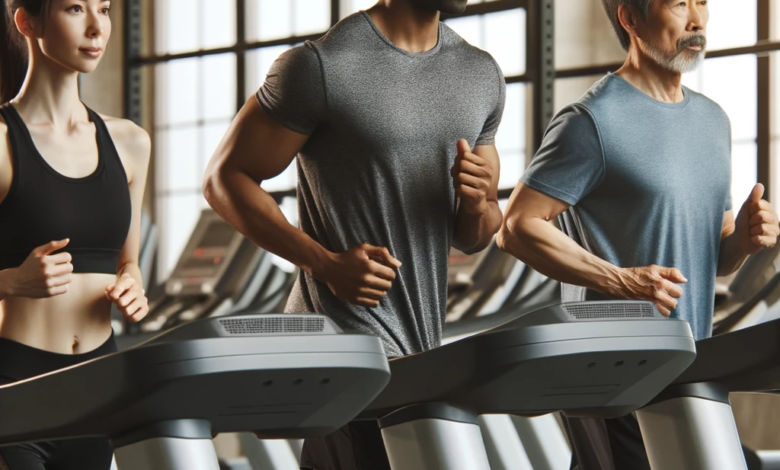
Dietary protein, while essential, is just one component in achieving optimal muscle adaptation through physical training. The additional protein needed beyond a regular diet is typically minimal, and many athletes already fulfill their daily protein needs. It’s crucial for athletes to realize that achieving their goals depends on a combination of consistent, structured training and a balanced diet that satisfies their energy requirements. External factors such as stress, excessive alcohol consumption, and lack of rest can significantly reduce the effectiveness of a well-designed diet and exercise plan. Despite this, the role of protein remains significant. Recent research has highlighted how dietary protein not only supports muscle growth (hypertrophy) and metabolic changes but also plays several key roles in sports performance. These include enhancing muscle mass and strength, aiding in metabolic adaptations (like increasing oxidative enzymes), preserving lean mass during rapid weight loss, and providing structural benefits to protein-rich non-muscle tissues such as tendons, ligaments, and bones.
Contemporary guidelines provide detailed information on daily protein requirements and how protein intake should be distributed. These guidelines take into account the changes in protein metabolism during and after exercise, integrating this data with broader nutritional strategies. This holistic approach underscores the importance of protein in an athlete’s diet, not just for muscle development but for overall physical health and performance.
During physical exercise, the body primarily relies on carbohydrates and fats as the main sources of energy for muscles. However, proteins also have a significant, though lesser, role in this process. They are directly oxidized in the muscles, especially when the body’s carbohydrate reserves are running low. This oxidation of proteins during exercise is a part of the body’s complex energy-production system.
Typically, the contribution of protein to the body’s total energy expenditure during exercise is relatively small, usually accounting for less than 5 to 10 percent. In more extreme cases, particularly during prolonged or high-intensity workouts, this percentage can increase to up to 15 percent. Several factors determine the extent to which protein is used for energy:
- Exercise Intensity: The more intense the workout, the more the body may rely on protein as a source of energy, especially when other fuel sources are diminished.
- Training Level: An individual’s training level also influences protein use. Experienced athletes may have more efficient energy utilization from carbohydrates and fats compared to those newer to intense physical training.
- Availability of Other Fuels: The body’s store of carbohydrates, specifically muscle glycogen, plays a crucial role. When glycogen stores are high, the body prefers to use carbohydrates for energy. As these stores deplete, the body begins to oxidize protein to a greater extent.
- Type of Exercise: Different forms of exercise affect protein oxidation rates differently. For example, during intense resistance training, less than 5 percent of energy comes from protein. However, during prolonged endurance exercises (lasting over 90 minutes), the usage of protein can increase significantly.
When muscle glycogen levels are low, the body activates processes like gluconeogenesis and ketone production to maintain stable blood sugar levels, essential for fueling the nervous system. Gluconeogenesis involves creating new glucose from non-carbohydrate sources, including protein. In individuals following low-carbohydrate diets, ketone production becomes a more significant energy source when glucose is scarce.
As a result of these processes, when glycogen stores are depleted, there’s an increase in the oxidation of branched-chain amino acids (BCAAs) within the muscles, leading to a higher use of protein as a fuel source. This shift in energy production can lead to a decrease in exercise intensity, as the body’s ability to generate high levels of ATP (adenosine triphosphate, the energy currency of the cell) through gluconeogenesis and ketone production is not as efficient as through glycogen breakdown.
Gluconeogenesis:
A key gluconeogenic pathway is the glucose-alanine cycle. In this pathway, the amino acid alanine leaves the muscle to create new glucose in the liver, contributing to blood glucose levels. This occurs alongside muscle tissue oxidation of BCAAs, where their NH2 group is transferred to pyruvate in a process called transamination. The carbon skeletons from BCAAs then enter the TCA cycle in muscle cell mitochondria as intermediates, aiding ATP generation. Meanwhile, pyruvate from glycolysis binds with NH2 from BCAA deamination to form alanine, which can leave the muscle and travel to the liver. The liver then deaminates alanine to reform pyruvate, further metabolized to contribute to blood glucose or liver glycogen. Gluconeogenesis is a continuous process in human metabolism, increasing during prolonged exercise, especially when carbohydrate stores are low. The speed of new glucose creation depends on the availability of necessary enzymes, which can’t be produced fast enough to meet the demands of long endurance exercises. This can lead to hypoglycemia, often the main reason exercise stops during long events. While the glucose-alanine cycle is crucial in human metabolism and contributes to exercise metabolism, it’s more suited for non-exercise situations, like starvation, when key enzymes can be up-regulated to produce glucose at the expense of muscle protein.